Biochemistry of flavours in a sourdough bread
- Anushka Neyol
- Oct 12, 2022
- 5 min read
Among the different parameters that define bread quality, flavour is one of the most appreciated sensory attributes (Caul, 1972). In fact, the concept of bread flavour has been the most contentious of all the quality parameters (Cauvain, 2003).
More than 540 volatile compounds have been reported in bread, although only a relative small portion of the volatile compounds in bread contribute to the desirable aroma properties (Cho & Peterson, 2010). These compounds are called “aroma compounds” and they can be defined as the volatile compounds that are likely to be sensed when the bread is eaten. Concretely, volatile compounds with OAVs higher than 1 are considered aroma compounds, since their concentration in bread is higher than the corresponding OT.
Figure 5 compresses the 28 volatile compounds most cited in the literature classified by their biological origin.
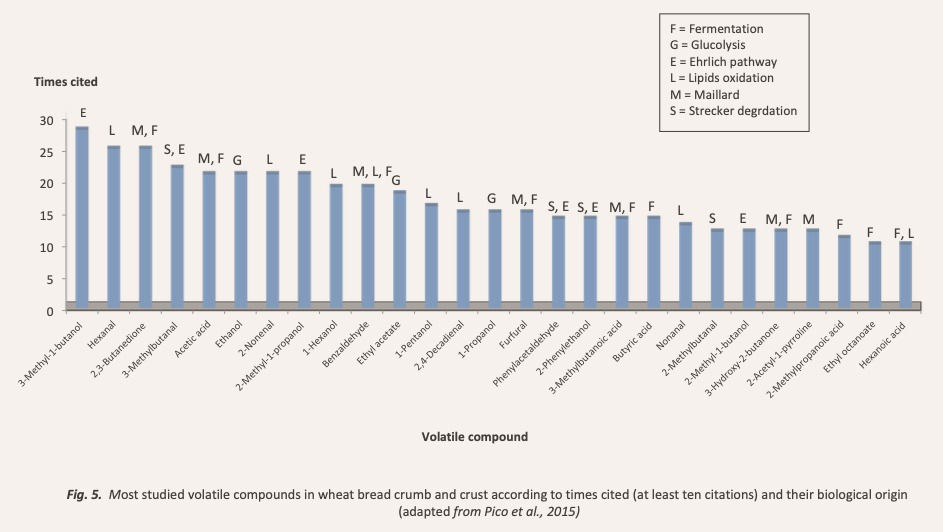
Fermentation produces alcohols, aldehydes, acids, esters and ketones (Bianchi, Careri, Chiavaro, Musci, & Vittadini, 2008; Cho & Peterson, 2010; Martínez-Anaya, 1996) by the action over the sugars of yeasts, above all Saccharomyces Cerevisiae, and lactic acid bacteria (LAB), highlighting these of the genus Lactobacillus and Kluyveromyces (Pico et al., 2015).
Saccharomyces Cerevisiae is naturally present in the flour in small concentrations, but it is usually added to the dough in order to promote the fermentation against the lipid oxidation; when there is no addition of yeast, oxygen is more available and it is used by lipoxygenase enzymes to generate aldehydes from the oxidation of lipids usually with off-flavours (Poinot et al., 2008).
However, when S. Cerevisiae is added, it uses the oxygen in an aerobic process to grow during kneading, encouraging the generation of volatile compounds from fermentation (anaerobic process). In fact, Frasse, Lambert, Richard-Molard, & Chiron (1993) reported that if there was no external addition of S. cerevisiae, the major aroma impact compounds in dough were 2,4-(E,E)- decadienal, 2-(E)-nonenal, from lipid oxidation, and methional, from Ehrlich pathway. However, when S. cerevisiae was added to the dough, the major aroma impact compounds were 3-methyl-1-butanol, 2-methyl-1-butanol, 2,3- butanedione, methional and 2-phenylethanol, all of them from fermentation.
95% of these sugars are fermented by S.Cerevisiae into ethanol and carbon dioxide, while the remaining 5% participate in secondary fermentation reactions, namely glycolisis of pyruvic acid (Czerny & Schieberle, 2002) and the Ehrlich pathway (Birch, Petersen, Arneborg, et al., 2013), both processes being summarised in Figure 6.
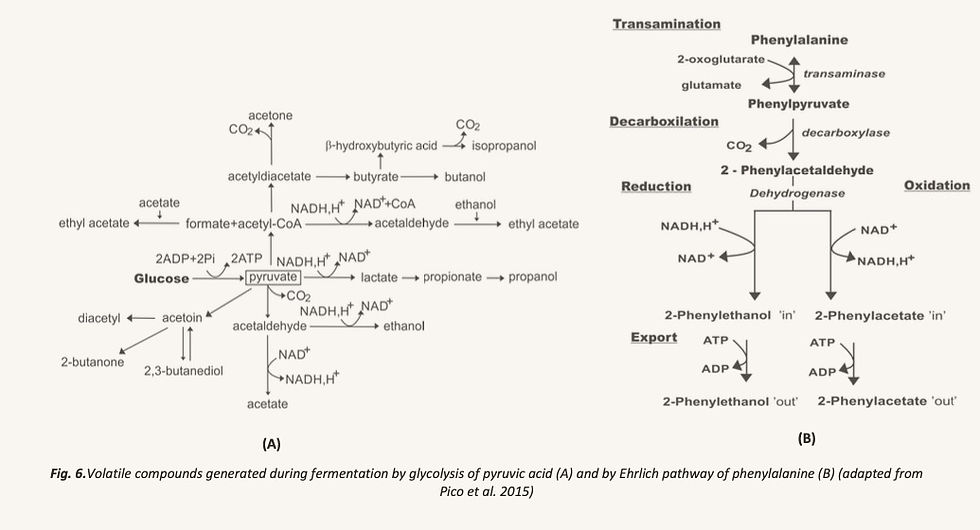
Short chain alcohols, short chain fatty acids, esters and carbonyl compounds are generated during the glycolisis of pyruvic acid (Drapon & Richard- Molar, 1979), while higher molecular weight alcohols are obtained from the Ehrlich pathway through the transamination, decarboxilation and reduction/ oxidation of initial amino acids. In this way, (i) 3-methylbutanal, 3-methyl-1-butanol and 3- methylbutanoate derive from leucine; (ii) 2-methylpropanal, 2-methylpropanol and 2-methylpropanoate from valine; (iii) 2-methylbutanal, 2-methyl-1-butanol and 2- methylbutanoate from isoleucine; (iv) 2-phenylethanal, 2-phenylethanol and 2- phenylethanoate from phenylalanine; (v) methional, methionol and 3-(methylthio)- propanoate from methionine (Hazelwood et al., 2008).Finally, regarding the action of LABs, they are normally added to the recipe using sourdough (Plessas, Fisher, et al., 2008; Ur-Rehman et al., 2006).
The coexistence of yeast and LABs generates a greater number of volatile compounds than if only LABs were added (B. Hansen & Hansen, 1994). However, if the dough already contained yeasts, LABs do not significantly modify the volatile profile, just an increase in the short-chain organic acids (Martínez-Anaya, 1996). Naturally, the fermentation time and temperature would also affect the generation of volatile compounds (Cauvain, 1998; Wiggins, 1998; Zehentbauer & Grosch, 1998b).
Lipid oxidation includes the generation of aldehydes, alcohols, ketones and furans (Birch et al., 2014), through the transformation of polyunsaturated fatty acids (mainly linoleic and linolenic acids) in hydroperoxides, which are unstable and degrade during baking into a great number of volatile compounds (Pico et al., 2015), as it is shown in Figure 7. Lipoxygenases are the enzymes responsible of using the oxygen to transform the unsaturated fatty acids into volatile compounds, namely 2,4-(E,E)-decadienal, nonanal, 2-(E)-nonenal, octanal, heptanal, 1-pentanol, 1-hexanol, 1-octen-3-one or 2-pentylfuran (Birch et al., 2014; Pico et al., 2015). Therefore, the availability of the oxygen as well as the amount of polyunsaturated fatty acids in the flour, have been considered key factors. Moreover, the lipoxygenase activity differs between different flours, since rice and wheat present high lipoxygenase activities (Leenhardt et al., 2006; Wongdechsarekul & Kongkiattikajorn, 2010), while in quinoa flour it is almost negligible (Caussette, Kershaw, & Shelton, 1997).
Finally, the content of antioxidants, such as vitamin E and flavonoids, is also important in order to inhibit the lipoxygenase action (Pico, Hansen, & Petersen, 2017).
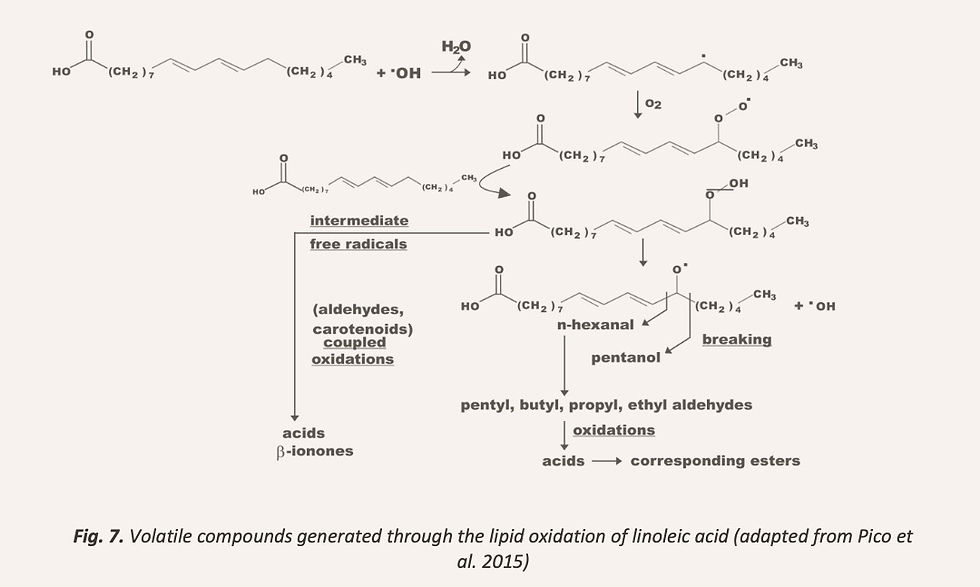
Maillard reactions refer to the chemical reactions between an amino acid and a reducing sugar, such as glucose and fructose, in the presence of temperatures between 110°C - 150°C (Onishi, Inoue, Araki, Iwabuchi, & Sagara, 2011a), leading to the formation of brown pigments (melanoidines) and a large number of volatile compounds (Pico et al., 2015), as can be noted in Figure 8. Thereby, Maillard reactions generate, principally, furans, pyrazines, pyrroles, pyrrolines, oxazoles, thiophenes, thiopyranes, thiazolines and sulphuric compounds in crust during baking (Bianchi et al., 2008; Bredie, Mottram, & Guy, 2002; Cho & Peterson, 2010).
The Strecker degradation is one of the main steps of Maillard reactions and it implies the reaction between amino acids and dehydroreductones to produce aldehydes with the same structure of the former amino acid (Guinet & Godon, 1996). Consequently, acetaldehyde comes from alanine, formaldehyde from glycine, glyoxal from serine, 2-hydroxypropanal from threonine (Rooney, Salem, & Johnson, 1967) and, also generated through the Ehrlich pathway, 2-methylpropanal from valine, 3-methylbutanal from leucine, 2-methylbutanal from isoleucine, phenylacetaldehyde from phenylalanine and methional from methionine (Martínez-Anaya, 1996; Rooney et al., 1967).

The rate of the Maillard reaction depends on the type of sugar, being xylose the most reactive, whilst the aroma profile relies on the type of amino acid (Kiely, Nowlin, & Moriarty, 1960). On the other hand, the temperature acts as a catalyst and the humidity of the oven favours the starch dextrinisation and generation of sugars for Maillard reaction (Guinet & Godon, 1996). 2-acetyl-1-pyrroline has been considered the key aroma compound of Maillard reaction, but 2-methylbutanal, 3- methylbutanal, methional (all three are also generated by the Ehrlich pathway), 3- methylbutyric acid, 2,3-butanedione (also from fermentation), 4-hydroxy-2,5- dimethyl-3(2H)-furanone and 2-methyl-propanal, were also important Maillard compounds due to their high OAV.
Special mention should be made to acrylamide, furan and 5-hydroxymethylfurfural, since they are known as potentially harmful compounds associated to mutagenic, carcinogenic and cytotoxic effects (Capuano & Fogliano, 2011). Although acrylamide is not very volatile (boiling point 241 °C), it is commonly present in bread, generated from asparagines degradation with reducing sugars (Mottram, Wedzicha & Dodson, 2002).
5-hydroxymethylfurfural can be generated by Maillard reactions and caramelisation, while furan is generated by lipid oxidation, thermal degradation of ascorbic acid and Maillard reactions (Rannou, Laroque, Renault, Prost, & Sérot, 2016).
Caramelisation is also a thermal reaction that takes place at temperatures higher than 150°C (Hadiyanto et al., 2007; Onishi et al., 2011a), when the sugars are heating above their melting point. The range of temperatures can be overlapped with the Maillard reaction and some volatile compounds can be generated by both sources, specially furan derivatives, such as furfural, 5-methyl-2-furaldehyde and furfuryl alcohol (Ait Ameur, Rega, Giampaoli, Trystram, & Birlouez-Aragon, 2008).
Furfural is generated by the reaction between phenylalanine and xylose (Nakama, Kim, Shinohara, & Omura, 2014) and 5-methyl-2-furaldehyde is derived from phenylalanine and rhamnose (Buera, Chirife, Resnik, & Wetzler, 1987). However, both furfural and 5-methyl-2-furaldehyde could be produced by pentose caramelisation (Ait Ameur et al., 2008). Furfuryl alcohol is the only mainly reported as a reduction product from furfural (Spillman, Pollnitz, Liacopoulos, Pardon, & Sefton, 1998).
Sources:
Wheat bread aroma compounds in crumb and crust: A review (Pico et al)
Analytical methods for volatile compounds in wheat bread (Pico et al)
We hope this was helpful, and you could learn something new about sourdough. If you have further questions and would love to know anything in specific, please carry on the discussion on our discord channel in #wheatywednesdays thread.
Comments